Action Potentials and Synapses
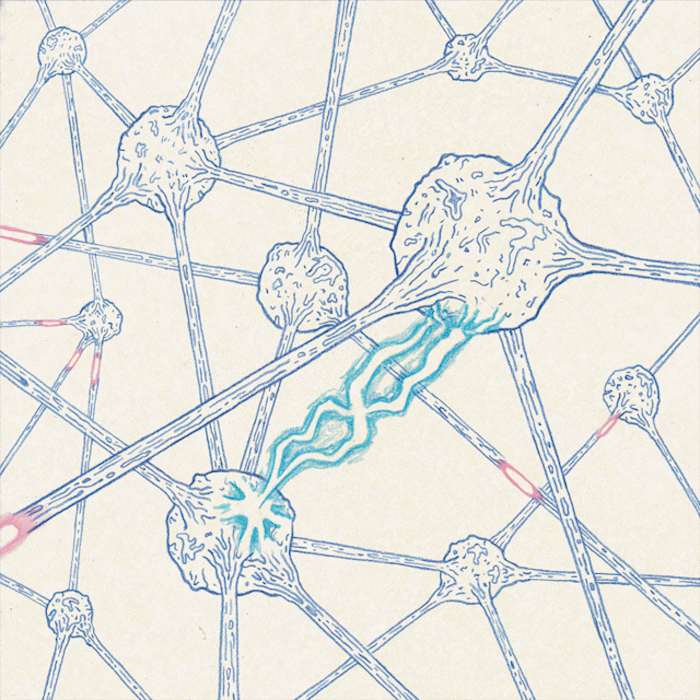
The anatomy of the neuron allows action potentials to propagate along the axon.
We begin by discussing what occurs once a depolarization arrives at the axon terminal, the synapse provides a mechanism for an action potential in one neuron to depolarize another neuron, giving the nervous system its information transmitting capability.We then review the structure and function of synapses and neurotransmitters, the chemical signals found at synapses. Finally, we use this information to reexamine the physiological basis of common nervous system activities.
The action potential, which is a stereotypic pattern of depolarization, is one of the most important features of how neurons work.
The voltage at which the action potential is triggered is called the threshold voltage. Unlike the graded potentials we looked at first, the action potential has a fixed amplitude and duration. It is an all-or-none event, and it happens the same way every time. . The action potential is caused by rapid and reversible changes in ionic permeabilities across the cell membrane. These changes reflect the activity of voltage-gated ion channels that are normally closed but open when the voltage across the cell membrane depolarizes. There are two types of voltage-gated channels, one selective for Na+ and another selective for K+.
These channels differ in two important ways.
- First, the Na+ channel responds very quickly to changes in membrane voltage, while the K+ voltage-gated channel responds more slowly.
- Second, the Na+ channel can stay open only for a relatively brief period of time—once it opens, it closes again regardless of the membrane potential. This property is called inactivation. The K+ channel does not inactivate; it stays open as long as the membrane is depolarized.
The action potential is the result of the activity of K+ and Na+ ion channels.
At the resting membrane potential, both the Na+ and K+ voltage-gated channels are closed and will not permit ions to pass. When the membrane begins to depolarize for any reason, the voltagegatedchannels begin to open. The Na+ channel opens quickly, however, while the K+ channel opens much more slowly. As a result, the permeability of Na+ increases dramatically and Na+ rushes into the cell. Na+ entering the cell depolarizes it further, increasing the number of open Na+ channels and, therefore, the amount of Na+ entering the cell, which depolarizes the cell even more. This positive feedback cycle continues until all the Na+ channels are open and Na+ permeability is at its maximum. This is why, once triggered, the action potential is an all-or-none event.
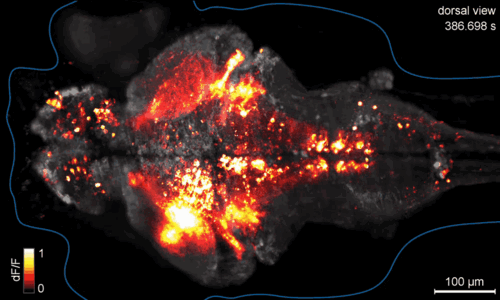
The membrane potential repolarizes as rapidly as it depolarized, for two reasons.
- First, the Na+ voltage-gated channels inactivate, decreasing the permeability of Na+ back to its normal resting level. There appear to be two protein “gates” in this channel—one that opens with depolarization and another that closes a short time afterwards.
- Second, the K+ voltage-gated channels begin to open. These channels are responding to depolarization, just like the Na+
channels but more slowly. The Na+ channels have already opened and started to close again by the time the K+ channels open. The opening of these channels increases the movement of K+ out of the cell, making the inside of the cell more negative.
When the cell membrane returns to its normal resting potential, the voltage that caused the K+ channels to open is gone, but they remain open for a while longer because of their slow response. This causes the membrane to undershoot its resting membrane potential (become more negative than normal). In a millisecond or two, the K+ channels close and the membrane potential returns to its normal resting level. After an action potential, there is a refractory period during which another action potential cannot be triggered. If the membrane is depolarized immediately following one action potential, another action
potential will not be triggered. The refractory period is caused primarily by the fact that the inactivation gates of the Na+ channels remain closed for a few milliseconds after the membrane has re-polarized. The refractory period sets the maximum rate at which a neuron can generate action potentials.
Action potentials must be able to propagate to be useful as signals.
The most important feature of membrane depolarization in nerve cells is that it can propagate, or move from one place to another along the axon. Action potentials propagate without any degradation; this ability enables the nervous system to transmit information over long distances. The anatomy of neurons lends itself to long-distance communication, and the action potential travels down the cellular “wire” of the axon in a simple fashion. If an action potential is triggered at one end of an axon, Na+ will rush into this area because its permeability is greatly increased in this location. This influx of Na+ not only depolarizes the region around open channels, but it also causes adjacent areas to depolarize as well. If an adjacent segment of the axon is depolarized beyond its threshold, voltage-gated channels will open up and trigger an action potential in that segment.. This process continues down the axon, like a row of standing dominoes falling over in sequence when the first domino in the row is tipped. However, propagation is really a continuous wave of depolarization down the axon, not a series of discrete events. As each section of an axon passes the threshold for an action potential, its response is just as strong as the first. Action potentials typically travel in only one direction in a neuron, they are initiated at the cell body and travel away from it down the axon to the axon terminal. This is because the wave of depolarization leaves refractory segments in its wake, which cannot be immediately depolarized.
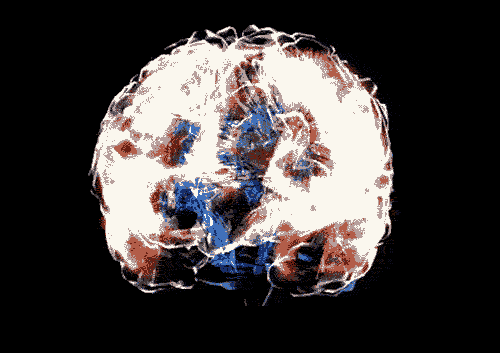
Synapses transfer depolarization between neurons.
Once triggered, an action potential travels down the axon to the axon terminal, which typically terminates on the body or dendrites of another cell. The point of contact between these two cells is called a synapse. The transmitting cell is called the presynaptic cell, and the receiving cell is called the postsynaptic cell. Synapses allow depolarization in one cell to pass to an adjacent cell. It seems reasonable, therefore, that synapses would have channels that allow ionic current to pass directly from pre-synaptic to post-synaptic cells.
Electrical synapses do have inter-cellular channels, called gap junctions, which form a continuous cytoplasmic connection between two cells. Electrical synapses enable the action potential to pass unimpeded from one cell to the next, but they are relatively uncommon in vertebrates. Chemical synapses are by far the most common type. Chemical synapses have a very narrow space between the membrane of the pre-synaptic cell’s axon terminal and the membrane of the post-synaptic cell. An action potential cannot cross this space; instead, it is the electrical signal converted into a chemical signal that crosses to the other cell, where it is converted back into an electrical signal.
Understanding the structure of chemical synapses is crucial to understanding their function.
The pre-synaptic cell membrane has a large number of membrane-bound vesicles, each of which contains a quantity of a chemical signal called a neurotransmitter. There are many different kinds of neurotransmitters. When the depolarization of the action potential reaches the presynaptic terminal, these vesicles move to the cell membrane, fuse with it, and release their contents into the narrow space between the two cells. The mechanism by which the vesicles release their contents involves a set of calcium (Ca++) voltage gated ion channels. The postsynaptic cell membrane is covered with transmembrane proteins that act as highly specific receptors for neurotransmitters. Each receptor will specifically bind only with a particular type of neurotransmitter.
There are two general kinds of neurotransmitter receptors.
In the first kind, the receptor molecule is itself an ion channel or part of an ion channel. When the neurotransmitter binds to the receptor, it causes a shape change in the channel. Because the neurotransmitter binds to the channel protein itself, this is called a directly gated channel.
The second kind of neurotransmitter receptors are indirectly gated. In these cases, the neurotransmitter binds to a receptor that activates a second messenger system.
In both cases, the effect of the neurotransmitter is to change ionic permeability on the post-synaptic membrane, which converts the chemical signal back to an electrical signal.
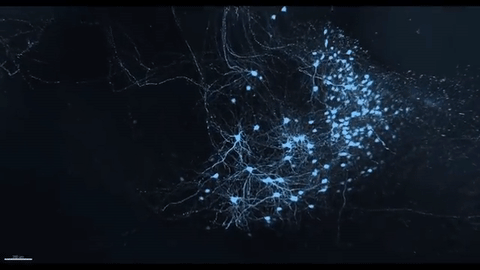
Some neurotransmitters may depolarize the post-synaptic membrane.
This brings the post-synaptic cell closer to its threshold; others may hyperpolarize the postsynaptic cell and make it less likely to fire an action potential. The effect a neurotransmitter has depends on which ion the receptor channel allows to pass and whether the neurotransmitter opens closed channels or closes open channels. Regardless of which effect a neurotransmitter has, enzymes or other mechanisms in the synaptic cleft quickly remove it after it is released; thus, the effect of one action potential reaching the pre-synaptic terminal is brief and precise.
Though we have not examined in detail the type of information neurons convey, they essentially function by changing the permeability of Na+ and K+ ions across cell membranes. The nervous system responses as “simple” as juggling or as complex as love, fear, or remembering a face all stem from the action of ion channels.
Consider this
- Why is it important that the strength of an action potential (i.e., the extent of depolarization) remain unchanged along the length of an axon?
- What kinds of proteins do you think are most likely affected by mood altering drugs?
Sources: 1
Image Sources: Giphy
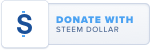
Thanks for Donating #steemit !
BTC: 1JQWNxziArfaCtxsP3tUQ4VSTBY9jresmt
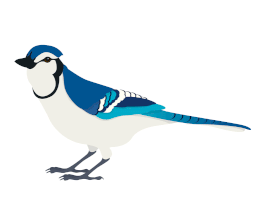
Great post about neurons. Are you also into signal transduction pathways? You'd probably able to explain it well. Thanks for your efforts. I appreciate it :-)
Greetings from @steemokto
This post has been ranked within the top 25 most undervalued posts in the first half of Dec 06. We estimate that this post is undervalued by $10.41 as compared to a scenario in which every voter had an equal say.
See the full rankings and details in The Daily Tribune: Dec 06 - Part I. You can also read about some of our methodology, data analysis and technical details in our initial post.
If you are the author and would prefer not to receive these comments, simply reply "Stop" to this comment.