Nuclear Fusion, the new hope
Nuclear fusion is a nuclear reaction in which two nuclei of light atoms, in general hydrogen and its isotopes (deuterium and tritium), join to form another heavier nucleus. Generally, this union is accompanied by the emission of particles (in the case of deuterium atomic nuclei a neutron is emitted). This nuclear fusion reaction releases or absorbs a large amount of energy in the form of gamma rays and also kinetic energy of the particles emitted. This large amount of energy allows the matter to enter the plasma state.
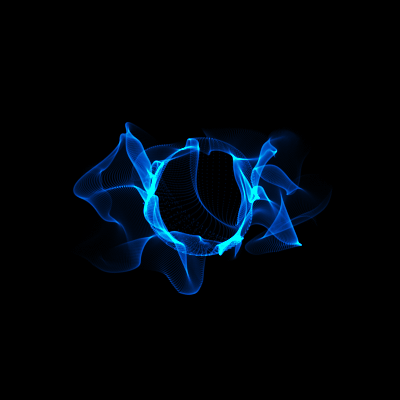
Nuclear fusion reactions can emit or absorb energy. If the nuclei to be fused have less mass than iron, energy is released. On the contrary, if the atomic nuclei that are fused are heavier than iron, the nuclear reaction absorbs energy.
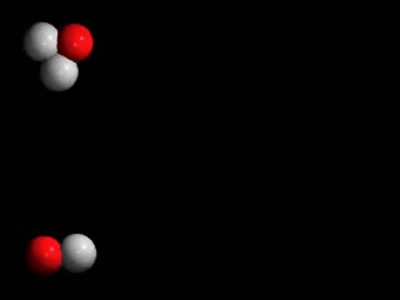
Do not confuse nuclear fusion with the fusion of the core of a reactor, which refers to the fusion of the reactor core of a nuclear power plant due to overheating caused by poor cooling. During the Fukushima nuclear accident, this expression was frequently used in the media.
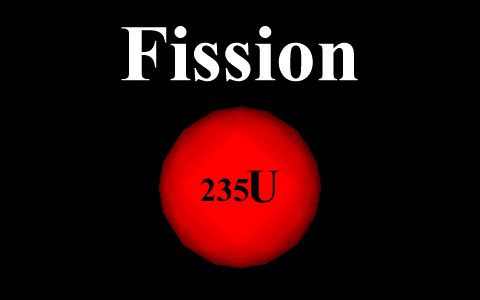
The stars, including the Sun, constantly experience nuclear fusion reactions. The light and heat that we perceive from the Sun are the resultst of these nuclear fusion reactions: hydrogen nuclei collide with each other and merge into a heavier nucleus of helium releasing an enormous amount of energy. The released energy reaches the Earth in the form of electromagnetic radiation.
The forces of gravity in the universe generate the perfect conditions for nuclear fusion.
Nuclear fusion reactions are also called thermonuclear reactions due to the high temperatures they experience. Inside the Sun, the temperature is close to 15 million degrees Celsius.
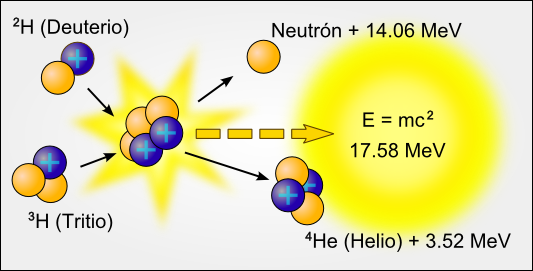
Technical requirements for nuclear fusion
To carry out nuclear fusion reactions, the following requirements must be met:
Achieve a very high temperature to separate the electrons from the nucleus and that it comes close to another by overcoming the electrostatic repulsive forces. The gaseous mass composed of free electrons and highly ionized atoms is called plasma. Confinement is necessary to keep the plasma at an elevated temperature for a minimum of time. Plasma density sufficient for the nuclei to be close to each other and generate reactions of nuclear fusion.
Confinement for nuclear fusion
Conventional confinements that are used in nuclear fission reactors are not possible due to the high temperatures of the plasma they must support. For this reason, two important methods of confinement have been developed:
Nuclear fusion by inertial confinement (FCI): It consists of creating a medium so dense that the particles have almost no chance of escaping without colliding with each other. A small sphere composed of deuterium and tritium is struck by a laser beam, causing its implosion. Thus, it becomes hundreds of times denser and explodes under the effects of the nuclear fusion reaction.
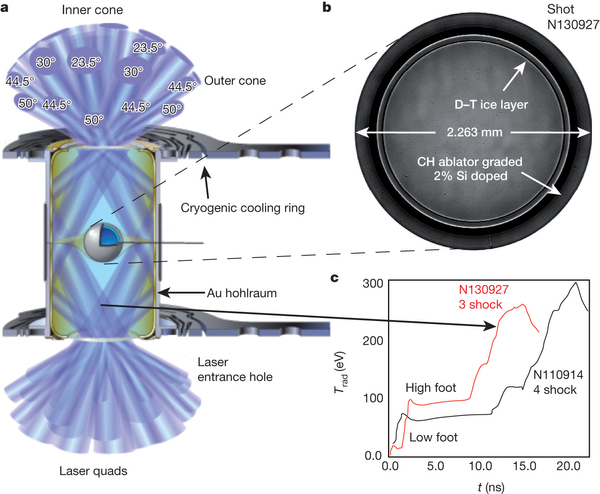
Magnetic confinement nuclear fusion (FCM): The electrically charged particles of the plasma are trapped in a reduced space by the action of a magnetic field. The most developed device is toroidal and is called Tokamak.
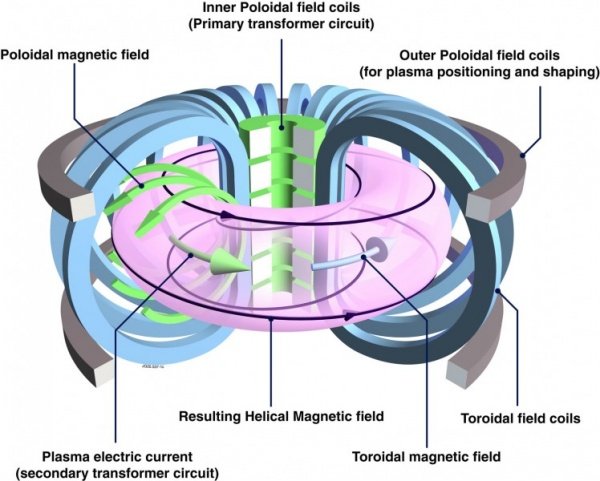
Nuclear fusion reactions
The atomic elements normally used in nuclear fusion reactions are hydrogen and its isotopes: Deuterium (D) and Tritium (T). The most important fusion reactions are:
D + T -> 4He + n + 17.6 MeV
Merging a Deuterium nucleus with a Tritium nucleus, a Helium nucleus consisting of two neutrons and two protons is obtained, releasing 1 neutron and 17.6 MeV of energy.
D + D -> 3He + n + 3.2 MeV
Merging two deuterium nuclei, you get a helium nucleus formed by a neutron and two protons, releasing a neutron and 3.2 MeV of energy.
D + D -> T + p + 4.03 MeV
Merging two Deuterium cores, you get a Tritium core, a proton and 4.03 MeV of energy.
For these reactions to take place, the nuclei must be supplied with the kinetic energy necessary for the nuclei to be fused to approach, thus overcoming the electrostatic repulsive forces. This requires heating the gas to very high temperatures, such as those that are supposed to take place in the center of the stars.
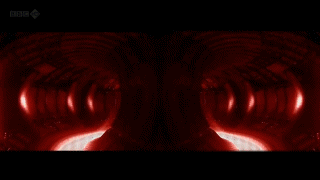.gif)
The requirement of any nuclear fusion reactor is to confine said plasma with temperature and density high enough and for just the right time, in order to allow sufficient nuclear fusion reactions to occur, preventing the particles from escaping, to obtain a net gain of energy. This energy gain depends on the energy needed to heat and confine the plasma being less than the energy released by the nuclear fusion reactions. In principle, for each milligram of deuterium-tritium, 335 MJ can be obtained.
Fuel used for nuclear fusion reactions
Light nuclei are required for nuclear fusion reactions. Basically, Deuterium and Tritium are used, which are two isotopes of hydrogen.
Deuterium is a stable isotope of hydrogen formed by a proton and a neutron. Its abundance in water is one atom for every 6,500 hydrogen atoms. This means that in seawater there is a concentration of 34 grams of deuterium per cubic meter of water. The energy content of deuterium is so high that the energy that can be obtained from the deuterium of one liter of seawater is equivalent to the energy that can be obtained from 250 liters of oil.
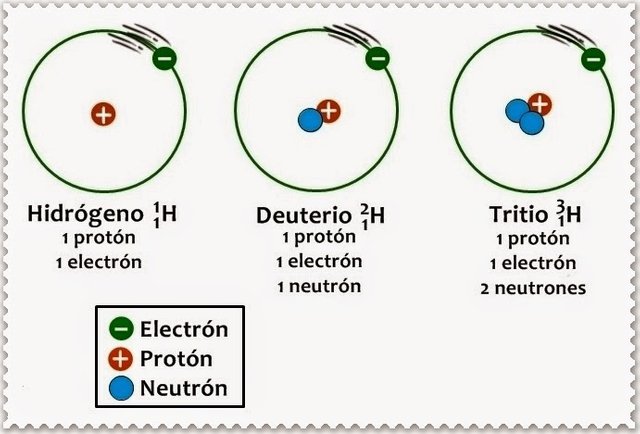
For this reason, taking into account that three-quarters of the planet is covered by water, nuclear fusion is considered an inexhaustible source of energy.
The other element used in nuclear fusion, Tritium, is the unstable or radioactive isotope of the hydrogen atom. It is composed of a proton and two neutrons and decays by beta emission relatively quickly. Although Tritium is scarce in nature, it can be generated by neutron capture reactions with the lithium isotopes. Lithium is an abundant material in the earth's crust and in the water of the sea.
Historical evolution and future projects on nuclear fusion
The origins of nuclear fusion are located around 1929 when Atkinson and Houtemans raised the possibility of obtaining energy from nuclear fusion reactions. However, the most important concepts of nuclear fusion and its real application were developed in 1942 with the works of H. Bethe, E. Fermi, R. Oppenheimer and E. Teller, among others. Through the Sherwood project, the first technological advances were carried out, which allowed the concept of magnetic confinement to be developed, obtaining the first designs: z-pinch, stellarator, and magnetic mirrors.
In 1961, J. Nuckolls (USA) and N. Basov (USSR) developed a technique by which nuclear fusion reactions could be obtained by high compressions caused by the transfer of energy. In this way, secret programs were developed in the USA and Russia. Subsequently, France joins this development, also secret. Other countries such as Germany, Japan, Italy and the USA (Rochester) developed open programs.
In 1965, Artsimovich presented the results of his research, at the "2nd Conference on Plasma and Controlled Fusion", on the TOKAMAK concept (Toroidal Kamera MagnetiK).
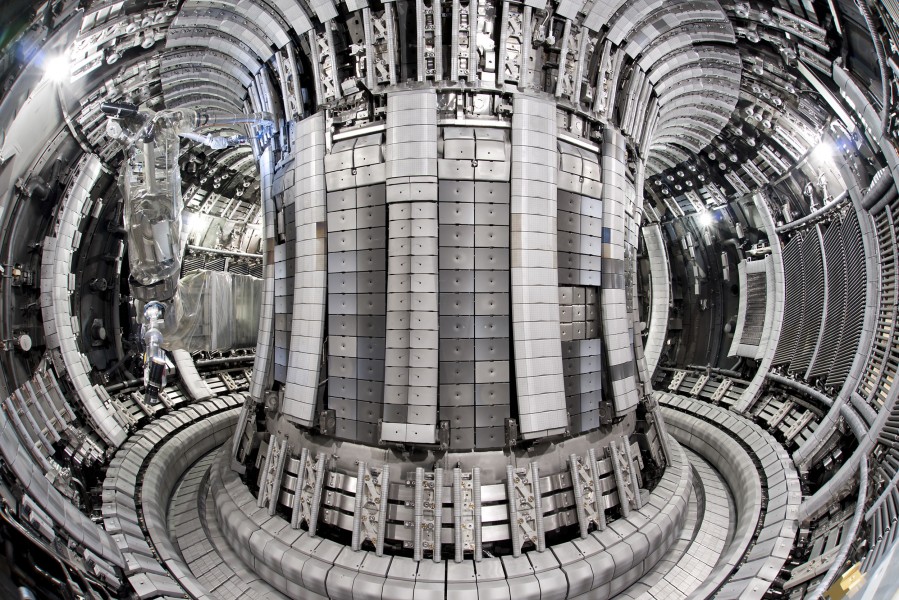
In the TOKAMAK concept, the magnetic field necessary to confine the plasma is the result of the combination of a toroidal field, a poloidal field, both created by toroidal coils, and a vertical field (created by a transformer). The plasma acts as a secondary of a transformer where the current that warms it is induced. A variable current intensity flows through the primary of the transformer.
In 1968, the Nobel Prize winner N. Basov, reported the obtaining of ignition temperatures and the production of neutrons in nuclear fusion reactions using lasers. From then on, it was possible to have a large number of devices under construction and operation under the TOKAMAK concept such as the following: TFR (France), T-4 and T-11 (USSR), ALCATOR and ORMAK (USA). Others such as the T-10 (USSR), PLT (USA), DITE (GB), ASEDX (RFA) and FRASCATI (EURATOM-Italy) began to be built.
In the 1970s, the first series of publications on FCI (Nuclear Fusion for Inertial Confinement) began to be produced. In the USA, the main researchers were Brueckner, Nuckolls, Kidder, and Clark. In Russia, Basov and his team got the most advanced experiment, reaching about 3 million neutrons in the implosion of CD2 spheres.
Based on this concept there are and have been many laser installations that have allowed advanced research on nuclear fusion. Of them, we can highlight NOVA (40 kJ, EUUU), OMEGA (30 kJ), GEKKO-XII (10 kJ, Japan), PHEBUS (3 kJ, France), VOLCAN (UK), ISKRA-5 (Russia).
Two large projects have been developed from these laser facilities to demonstrate high profits: National Ignition Facility (NIF) in the US and Laser Megajoule (LMJ) in France.
But the laser is not the only device capable of producing implosions, it is also observed that electrons and light and heavy ion beams are serious candidates for nuclear fusion by inertial confinement. The following projects with light ions are born: ANGARA and PROTO (Russia), PBFA-I and PBFA-II (USA).
In relation to heavy ions, in the absence of experiments, exact results could not be achieved, although certain predictions have been made by means of theoretical simulations such as those carried out in the HIDIF Project (Heavy Ion Design of Ignition Facility) sponsored by several European laboratories and institutes. and by the American Lawrence Berkeley Laboratory.
In the 90s, the facilities of the TOKAMAK type: JET (EURATOM), TFTR (USA) and JT-60 (Japan), allowed to obtain certain power. The first was the JET, which with a mixture of D (90%) and T (10%) achieved in 1991, a power of 1.7 MW. Subsequently, in 1993, the TFTR with a 50% DT mixture reached up to 6 MW, reaching temperatures of 30 keV. During the heating, 29 MW were used. Currently, the TFTR is closed. To date, up to 12 MW of power has been produced in controlled nuclear fusion reactions for more than one second (JET, 1997) and there is confidence that with current technological advances it is possible to reach the commercial range of hundreds of MW in a maintained way.
The experimental research in FCM (Nuclear Fusion by Magnetic Confinement) in Spain has been concentrated in the CIEMAT (Center for Energy, Environmental and Technological Research), dating back to 1983, the year in which the first nuclear fusion machine was put into operation, the Tokamak TJ-I.
From this moment, research has progressed steadily, and thus, in 1994, the first nuclear fusion device built entirely in Spain has launched: the Stellerator TJ-I upgrade, which was ceded in 1999 to the University of Kiel. enter into operation the TJ-II.
The TJ-II was a great scientific leap with respect to previous experiments considering one of the three most advanced stellarators in the world along with the German Wendelstein 7-AS of the Max Planck Institute in Munich and the Japanese LHD of the University of Nagoya.
For more information, visit this pages:
https://energia-nuclear.net/que-es-la-energia-nuclear/fusion-nuclear
https://www.csn.es/fusion-nuclear
newton.cnice.mec.es/materiales_didacticos/energia/fusion.htm
I know you listed the source but you just copy/pasted the translation. Ideally you only want to copy a little of it and then link to the source.
Copying/Pasting full texts without adding anything original is frowned upon by the community.
Some tips to share content and add value:
Repeated copy/paste posts could be considered spam. Spam is discouraged by the community, and may result in action from the cheetah bot.
Hello, thanks for the observation, I will be doing my best to improve, my work, again thanks.