Freezing-in and freezing-out dark matter
In my last post, I introduced an important concept connected to dark matter: the relic density of dark matter. This relic (from the ancient times) is roughly the density of dark matter that is left in the universe today.
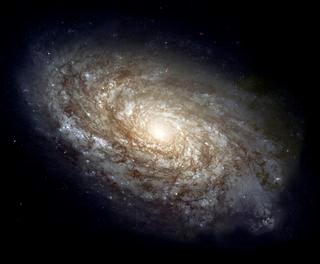
[image credits: Wikipedia]
Physicists have developed varied mechanisms that explain correctly the amount of dark matter that is observed today, and all relate the relic in one way or the other to the amount of dark matter of the early days of our universe.
Thermally freezing-out dark matter consists of one of these options, and I have largely detailed how this works in my previous post.
However, the correct relic density of dark matter can also be obtained by other means. For instance, instead of freezing-out, dark matter could freeze-in.
In any case, winter is coming, and everything must thus be frozen and dark ;)
This freeze-in is what I will talk about in this post, after having briefly summarized what is dark matter and how it freezes-out (so that the differences with the freeze-in case will be easier to point).
DARK MATTER IN A NUTSHELL
Before introducing the concepts of freezing-out and freezing-in dark matter, let me briefly recall what dark matter is. It consists of a type of matter that does not interact electromagnetically, which is the reason why it is called dark, and that interacts gravitationally, which is the reason why it is called matter.
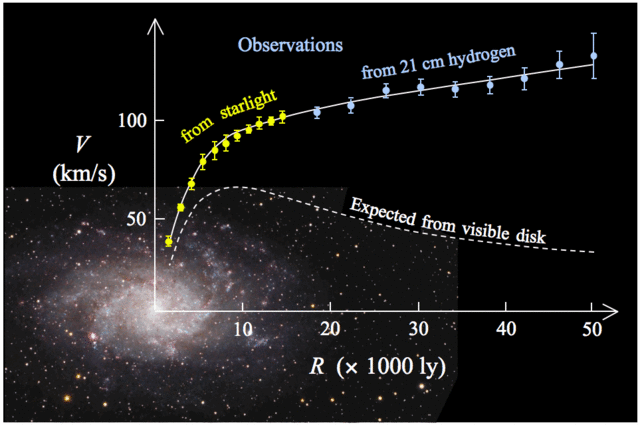
[image credits: Wikipedia]
Dark matter has been introduced almost 85 years ago to explain the dependence of the velocity of distant galaxies on the distance.
With the help of classical mechanics, it was shown that some invisible mass was required, so that one could have enough gravitational interactions to get an agreement between theory and experiment.
Without this invisible mass, it is indeed clear that the predictions (the dashed line on the left) disagree with data (the yellow and blue markers).
This invisible mass is what is called dark matter.
Furthermore, we know today that dark matter is also required to explain many many many (many?) observations, and this is why it is considered so seriously by physicists.
The only missing item on the agenda consists of its direct detection, at least for now.
FREEZING-OUT DARK MATTER
Before detailing how dark matter could freeze-in, let me recall what it does when it freezes-out. We can start from the figure of my older post, on which I have added colors to make it more visual below.
During the history of the universe, dark matter particles can undergo two reactions. Pairs of dark matter particles can be created from the collision of lighter particles (that are accelerated, like at the LHC, as the universe is super hot) and pairs of dark matter can be destroyed to give back pairs of lighter particles of the Standard Model.
In short, this is the same reaction that occurs from the left to the right and from the right to the left.
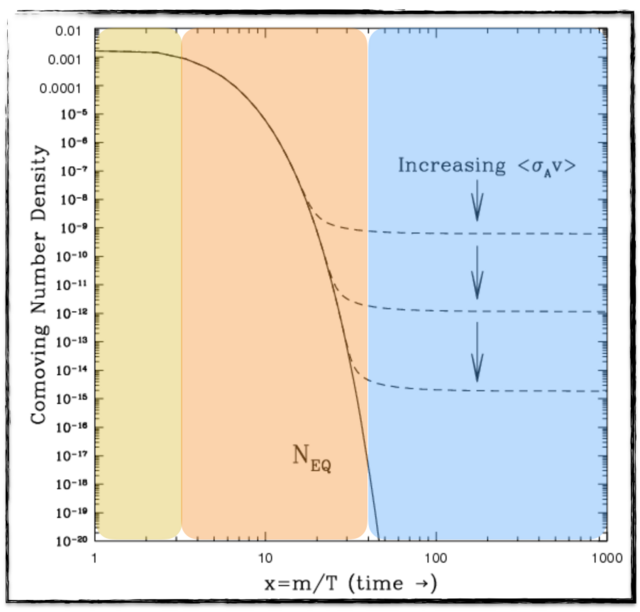
[image credits: homemade from Inspire]
At the origins of the universe (the yellow region on the figure), both reactions occur at the same rate.
The amount of dark matter that is created by the first reaction is thus equal to the amount of dark matter that is annihilated by the second reaction, so that the density of dark matter (that is what is represented on the y-axis of the figure) is constant.
The universe is however in expansion and its temperature goes down. As a result, lighter particles are at some point not energetic enough to create any new pair of dark matter particles, and one is left with a single reaction. Dark matter annihilates.
As a consequence, the density of dark matter goes down. This corresponds to the orange zone of the figure.
Finally, the universe continuing its expansion, dark matter starts to be too diluted to be able to annihilate. A single dark matter particle cannot find any neighbour anymore to annihilate together.
As a consequence, both reactions have stopped, and the dark matter density is constant, as on the blue region of the curve. This constant corresponds to the density of dark matter observed today.
FREEZING-IN DARK MATTER
In the freeze-out case, we have seen that one starts with a large amount of dark matter, that this amount is reduced during the history of the universe before getting constant at some point by virtue of a too cold and too expanded universe.
This is not the only way to produce the right amount of dark matter. A freeze-in mechanism is also possible, where one starts with very little dark matter at the time of the big-bang.
In the freeze-in context, the dark matter particle is a very feebly interacting guy that interacts very little with the other particles in the early universe.
In other words, dark matter is decoupled from the cosmic soup that originated from the big bang. It lives its life on its own, somehow.
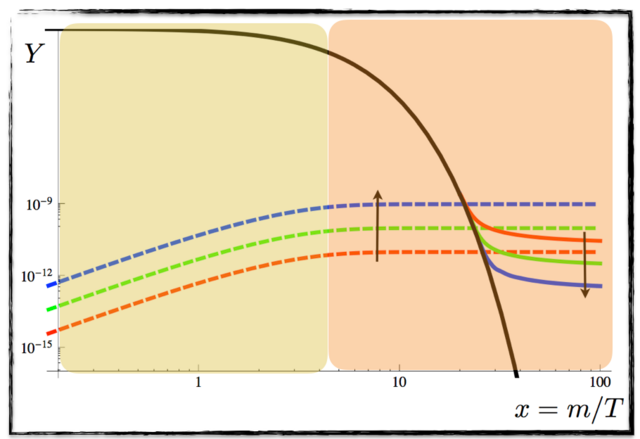
[image credits: homemade from arXiv]
Once in a while, additional dark matter particles can however produced, either through collisions of other particles or via the decay of heavier particles.
Consequently, the density of dark matter increases more and more with time. This is illustrated by the yellow region on the figure.
We need here to check the dashed lines and forget about the solid lines that represent the usual freeze-out case.
At some point, the universe will have already cooled down enough, so that there is not enough energy to produce more dark matter, as in the freeze-out case by the way.
The density of dark matter thus becomes constant (the orange area on the figure).
TAKE HOME MESSAGE
In this post, I came back to this freeze-out mechanism and re-discuss (hopefully more clearly) this mechanism that can successfully explain the amount of dark matter that is observed today. On the other hand, dark matter can also freeze-in instead of freezing-out.
The freeze-in mechanism can be seen as the opposite of the freeze-out mechanism. We start from almost no dark matter in the early days of the universe, and one slowly and slowly gets to the quantity of dark matter that is observed today. On the other hand, in the freeze-out case, one starts with a lot of dark matter in the early days of the universe, and dark matter is then annihilated until we get the right amount of dark matter observed today.
The only missing item on this dark matter agenda is of course its direct observation. Even if we have no direct observation of dark matter today, we must keep in mind that the set of indirect evidences is pretty large. And dark matter can be trickier that we think it is: there are still theoretically motivated ways for it to escape detection. However, the future experiments will definitely close this gap :)
This is, in my opinion, one of the things that make particle physics exciting.
For more discussion on this topic (or anything related to science), please join us on steemSTEM. SteemSTEM is a community driven project which seeks to promote well written/informative Science Technology Engineering and Mathematics postings on Steemit. More information can be found on the @steemstem blog.
We are currently consolidating the steemSTEM curation trail by different means. In the case where you would be interested, please contact us on the chat.
Thank you for the selection :)
Your two posts are really inspiring, thanks for sharing your knowledge on this fascinating subject with the community!
The two hypothesis are quite attractive. However, I am wondering about the x axis… What is m/T ? I suppose it is related to time in some way. So when would the transitions occur (2 for the freeze out, 1 for the freeze in)?
Another more in depth question:
In your opinion, what factor(s) could decide which of the two scenarios should be favored? I suppose that the dark matter genesis would occurre at the same time as the genesis of quarks and gluon, condensing from the huge PE of the inflaton field, once the inflation had stopped.
(ll the inflaton field KE had been converted to PE during the inflation phase, leading to energy condensation into particles when the inflation stopped. If I remember well, I think this is called the re-ionization phase , although I find this term confusing… )
Within this model, the origin of the x axis would be around t = 10^-32 seconds. In conclusion, what would decide if the initial quantities of dark matter were small or not vs. standard matter and antimatter particles.
And finally, do we have an idea of the energies required to reach conditions for dark matter genesis? Will it ever be within our reach?
Lots of questions here, sorry, but it’s really a fascinating topic. :-)
I cannot answer everything there... But I will try. Let's further discuss :)
This is the dark matter mass over temperature. As the temperature of the universe decreases with time, this is give or take the time, but the conversion is not immediate. However, as the temperature at the time of the big bang is finite, this defines the origins of the x-axis.
The only way to know would (I think) be to observe dark matter and study its properties. Knowing its mass and how it interacts with the Standard Model will be helpful. As long as this is not realized, I am afraid no one can tell. Both scenarios are equally plausible. I recall that the main difference between them is that in the freeze-out case, dark matter is in thermal equilibrium with the quark and gluon plasma, where in the freeze-in case, it is decoupled.
I am not too sure to see how the reionization plays a role here, but I may not have got the question correctly. And I am also not too sure about what you mean by quark, gluon and dark matter genesis? Sorry... Here we need to discuss more, I guess :)
Well , I understood that particles were created during the re-ionization phase (just as the Inflation ceased): Some of the potential energy of the inflaton field was converted to particles (Basically quarks and gluons). This is what I mean by quark and gluon genesis.
I was wondering if dark matter could have been created in the same process, or if it would have been the decay of the heaviest quarks + energetic collisions of quarks that could have created it, or a combination of both.
And in the end, if calculations based on the various inflaton field models could predict which of the freeze-out or freeze-in model would be favored (i.e., determining the relative quantity of Dark Matter created vs. normal matter).
Of course, these questions are only valid if we assume the inflationary models to hold some truth...
I guess that this is a very model dependent question and depending on the theory, you could have one or the other. For instance, in freeze-in model, there is no dark matter in the early days and it appears progressively. In freeze-out model, it could (should) be connected to inflation somehow.
Some could probably, I would say. But this is not easy (as one would try to solve many questions simultaneously).
Freeze out and freeze in, both amazing concepts are well explained here in your thought provoking post.
In Dirac's Large Number Hypothesis, he had the ratio of the total mass of the Universe divided by the mass of a proton is of the order of 1078. Do you know if he included dark matter and dark energy in the number he used for the total mass of the universe?
What do you think the total mass of the universe is?
Does the total mass (or total energy) of the universe increase, decrease or remain constant over the eons?
Thanks for your comment!
First, Dirac Large Number Hypothesis is a coincidence that Dirac observed and that is not really considered in today's physics. Just to point down this before starting ;)
The coincidence was recasted in terms of time (the age of the universe) and the ratio of the electromagnetic and gravitational interaction strengths between a proton and an electron. Therefore: no dark matter was necessary :)
We know that the universe is flat, so that its total energy density equals 9.9 x 10-30 g/cm3. Given that the observable universe is of about 14 billions light-years, then we get our answer (summing over both the luminous and dark stuff) :)
However, when you try to disentangle the various components (radiation, mass, dark energy, etc...), those vary with time.
Thank you for your wisdom.
The observable universe being 14 billion light-years means that we are observing stars that are about 14 billion years old. But 14 billion years ago the expanding universe was much much smaller. So what we are observing is stars in a tiny universe where the distance the light traveled to reach us is much less than 14 billion light-years, meaning that the light is much younger than 14 billion years old. It gets complicated. Do you know if there is a simple equation that explains this?
I am not so sure to follow the question... Do you mind rephrasing? :D
Sorry for the delay, I think I've been in a Spacetime warp right here on Earth
;-)
OK, here goes...
The light we see from the edge of our universe was emitted almost 14 billion years ago. I don't think the universe had a radius of almost 14 billion light years back when the light was emitted.
Spacetime been expanding for all that time, so that the distance to the farthest galaxies that emitted the light was much smaller when the light was emitted.
What was the approx. distance to the farthest galaxies when they emitted their light?
Do you know if there is a simple equation that clarifies the relationship between the age of the light and the distance it traveled in an expanding universe, maybe something like this?
A c = D = D0 S(t)
A = Age of the light
c = speed of light
D = Distance light traveled
D0 = Distance of light source at time of emission
S(t) = Spacetime expansion factor as a function of time
This is why we usually talk about the observable universe. This one has a radius of 14 billions light years. We however do not know what lies beyond it.
You may want to read about 'comoving distances' Does it help?
Thanks. Looking on wikipedia, comoving distances explains it, not as simple as I was guessing though.
With the universe, nothing is never really simple :p
What of the model where a dark matter particular is also its antiparticle, does it also need a neighbour to annihilate?
You always need two guys. It could be itself (if it is its own antiparticle) or the antiparticle (in the other case).
Or how about a guy and a gal? ;D
Like maybe before the big bang there was just 2 little particles, let's say, one electron and one positron, in empty space. Over the eons, they eventually collided. Since space was empty, velocity had no meaning. Only relative velocity had meaning (just like today). When they finally collided, their relative velocity was so near to the speed of light that their relative momentum carried enough energy E = pc (the rest mass portion of the 2 particles is so small that it can be ignored) that the single matter/antimatter annihilation explosion was the Big Bang.
Thought provoking posts get my imagination stimulated.
Why not :)
In my case, I don't really consider what could happen before the big bang, as the theory we know today ceases to be valid and there is actually no known way to probe that. Of course, others are thinking about options and it is good (in the same way one single person cannot take care of everything). ^^
The Freeze-in scenario looks more plausible as we could see in the LHC that heavier particles decays to dark matter. So maybe there were only visible matter at the beginning and the high temperature and collision created dark matter.
Also, it is funny of you to put a wiki link to the definition of many XD
Really nice article :)
Thanks for your comment :)
Freezing-out dark matter is still very very viable, even if not any dark matter particle has been found at the LHC so far. The LHC has excluded the most naive scenarios, but there are much more interesting models. Which is why anything non standard but theoretically motivated is interesting (we need to stay open-minded).
In fact, there is nothing that tells us that dark matter will be found at the LHC.
There is always a couple of unrelated links in my articles, for the fun ;)
Hey @lemouth long time no see ^^
I'm curious, are those freezing scenarios based on the hypothesis of Dark Matter being weakly interacting massive particles (WIMPs) and our knowledge of weak interaction or is this a more specific property?
Indeed. it was a long time :)
We are talking about feeble interactions that are many orders of magnitude weaker than the weak interactions. WIMPs are in fact thermal relics (they were in equilibrium with the thernal bath in the early universe) whilst FIMPS (feebly interacting massive particles) are never reaching this thermal equilibrium. They are totally decoupled.
In short, a WIMP will freeze-out no matter what.
Very interesting. I never heard of this freeze-in case but, well, I am not a particle- or an astro-physicist.
Do you maybe know when the density became constant? Cause if it was after the first galaxies came to life, then maybe we could see (indirectly) much smaller or larger quantities of dark matter in very old galaxies.
It really depends on the exact model (the mass of the dark matter particle and the strength of its interactions). But in any case, it is well before the formation of any star or galaxy (we are still talking about trillions of Kelvin).
So is there any way to experimentally confirm which model is correct. If any...
For the moment, everything is viable. The dark matter experiments are doing better and better, and the improvements of the last decade allow us to hope for the best for the next decade. But for the moment, there is still room for many things (which is why it is an exciting time ^^ ).
I am waiting for that time where it's observation won't be the way it is today without much of the theories (and counter theories) being thrown about. Particle physics is an interesting part of physics which one Prof almost seemed transported to somewhere not here when teaching it :)
The present time is really exciting. We have a lot of questions to which we look for an answer. The most naive approaches that were pursued in the past yields null results in terms of the experiment, so that one must start thinking more deeply about how to answer the questions. All those novels developments have now (and in the future) to be tested. At the same time, in parallel to the theory developments, the experiment is becoming more and more precise. These two things together help me (with other things in fact :p) to wake up with a smile every morning :)
The future would definitely be interesting, can't wait to see a novel approach break some serious boundaries and yield some awesome results.
Same for me:)
Oh yes!
Korea had some good effect on you ! more time to write posts ;)
I am not so sure that I have more time. I am hardly managing to write those days. I am happy to see that you have the impression I have not disappeared in the shadow ;)
The interested reader might also want to become an interested viewer of some mind-boggling facts about dark matter by visiting the following YouTube channel:
I've watched almost all of his videos. I recommend spending some time browsing through his videos. One of my favorites, though not related to this post, is about him explaining how the universe is much larger than we think it is.
I don't have the time to watch the videos (I rarely watch any video at all). I am the kind of person who prefers reading :)
Concerning this topic: dark matter is not antimatter and has never been produced (we are still at the level of trying to detect it). You are probably confused between both of these.
Is it? The (observable I suppose) universe has the size we calculated it has, no?
Sorry! You're right. I did confuse the two. I'm kind of like you because I do prefer reading to watching videos. However, this is one of those very few YouTube channels that I do actually watch sometimes.
The universe is much larger than the human mind can comprehend. For example, I think I remember one of my professors telling me that something to the order of 10^40 is more than however many atoms that we have in the universe. The human mind cannot fathom such things. That's what I think they're trying to convey.
Ah ok. There are indeed things we feel being like this or that. The universe may be one of these. However, feeling is different from having an actual theory and making predictions.
In fact, to rephrase it, our own feelings are by no way any scientific proof. It is not because we feel that something is like this or that, that it is actually like this or that :)