The Neurodevelopmental Toxicity of Fluoride: Focusing on Intelligence [Review by @SamoaSilk]
It's that time of year again y'all. Seems like once the calendar flips to September all I can think about is Halloween. Now as an adult, (aside from costumes, getting drunk, fucking shit up, etc.) the holiday conjures thoughts of rotting teeth and a heavy reinforcement of brushing habits. So, in the spirit of the holiday - I figured why not post some research considerably relevant to the prevention of dental carries resulting from the high sugar intake on October 31st. Below is some work I through together; please disregard any typos- there shouldn't be any, but maybe there's one I missed.
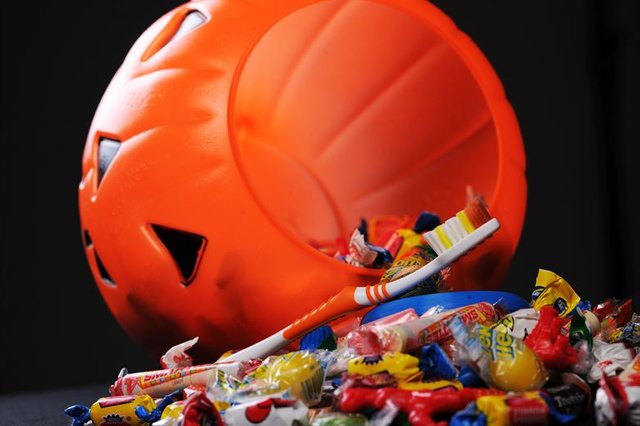
The Neurodevelopmental Toxicity of Fluoride on Intelligence
Fluoridated water supplies throughout the world are beginning to pose adverse effects on neurodevelopmental health among consumers. Today, fluoridation continues to expand across the United States, presenting a threat to hippocampal brain development within citizens, primarily children under the age of eight. Fluoride was recently reclassified as a chemical leading to developmental neurotoxicity, deemed toxic to the developing mammalian nervous system. Despite this reclassification, the U.S. Public Health Service Department has announced that the range of optimal fluoride concentrations in drinking water will increase dramatically by the year 2020.
Fluoride is the ionic form of the element fluorine and is the 13th most abundant element in the earth's crust. Chemically, fluoride is negatively charged and combines with positive ions to form stable compounds such as calcium fluoride or sodium fluoride. Such fluorides are released into the environment naturally in the form of both water and air. Generally, when the term fluoride is mentioned, thoughts associated with calcified tissues (i.e., bones and teeth) are provoked. This is likely due to fluoride’s high affinity for calcium, enabling its ability to inhibit or even reverse the initiation and progression of dental caries, otherwise known as tooth decay. Caries are an infectious, multifactorial disease afflicting most persons in industrialized countries and some developing countries. Today, all U.S. residents are exposed to fluoride to some degree, and widespread use of fluoride has been a major factor in the decline in the national prevalence and severity of dental caries. Although this decline is a major public health achievement, recent speculations have begun to surface after the release of several research studies conducting the safety and efficacy of ingesting this chemical. In fact, according to research published in Lancet Neurology, the highly prevalent chemical was reclassified as a developmental neurotoxin by the Harvard School of Public Health as of March 2014 (Grandjean & Landrigan, 2014). Fluoride is now believed to pose adverse effects during child neurodevelopment, influencing hippocampal regions associated with intelligence. This proclamation, however, wasn’t the first. The United States Environmental Protection Agency (EPA) screens and prioritizes chemicals for developmental neurotoxicity, and in turn assembles a list of chemicals that are toxic to the developing mammalian nervous system. The chemicals are assigned to one of three groups based upon the strength of evidence for neurotoxicity: no evidence, minimal evidence, and substantial evidence. Fluoride has been categorized as a developmental neurotoxin due to substantial evidence as of 2009 (Mundy, 2009).
Fluoride and Neurodevelopmental Disorders
Fluoridation
As previously mentioned, fluoride is freely available in nature; it can be found in the atmosphere, deriving from the dusts of fluoride-containing soils, gaseous industrial pollution, or from volcanic activity, as well as in fruit, vegetables and various other crops. Despite these common environmental producers, the majority of naturally occurring fluoride is found in bodies of water. Pools of water surrounded by fluoride-rich sediment can contain fluoride concentrations reaching as high as 50mg/liter when located near geothermal or volcanic activity. Contrary, other existing bodies of water contain significantly lower concentrations; seawater, for example, tends to have fluoride concentrations between 1.2-1.5 mg/liter. Surface waters, however, have the lowest levels of fluoride generally ranging from 0.01-0.3 mg/liter or relatively speaking, .01-.03 parts per million (ppm) (ADA, 2014).
In 1991, the U.S. Center for Disease Control released a Public Health Service report on fluoride disclosing the first act of fluoridation. The report states that in 1940, U.S. scientists discovered that people inhabiting geographical locations native to water sources that contained naturally occurring fluoride levels of approximately 1 part fluoride per million parts water or greater (>1.0 ppm) had fewer dental caries than people who lived relied on drinking water with lower levels (Centers for Disease Control, 1991). As a result of this discovery, Grand Rapids, Michigan adjusted the fluoride content of its water supply to 1.0 ppm just five years later, earning the title of the first city to implement community water fluoridation in the United States.
Throughout the late 1940s, the U.S. Public Health Service (PHS) developed recommendations regarding fluoride concentrations in public water supplies with the assumption that drinking water would be the major source of fluoride consumption by U.S. residents (Centers for Disease Control, 1991). The success of water fluoridation in preventing and controlling dental caries ultimately encouraged the development of fluoride-containing products, including toothpaste, mouth rinse, dietary supplements, and professionally applied or prescribed gel, foam, or varnish. In addition, agricultural products constitute for a small percentage of fluoride intake when processed with fluoridated water. Thus, U.S. residents have exponentially more sources of fluoride available now than 50 years ago.
Neurodevelopmental Disorders
With what seems to be an overabundance of government mandated fluoride consumption within the United States, health experts have been incapable of concurring whether fluoride in drinking water may be toxic to the developing human brain.
Currently, disorders of neurobehavioural development affect 10–15% of all births, and prevalence rates of autism spectrum disorder and attention-deficit hyperactivity disorder seem to be increasing not only in the states, but also worldwide.
Currently, the presence of neurodevelopmental disorders is increasing, and the cause of this global pandemic is only partially understood. Although genetic factors play a role, they cannot explain recent increases in reported prevalence; recent studies have shown that genetic factors, including recently discovered genes, can only be held accountable for roughly 30–40% of all cases of neurodevelopmental disorders (Grandjean & Landrigan, 2014). Therefore, it could be that non-genetic, environmental exposures are involved in causation, and in some cases are associated by interacting with genetically inherited predispositions.
Evidence exists suggesting that the dissemination of industrial chemicals in the environment can largely contribute to what may be considered the silent global pandemic of neurodevelopmental toxicity (Heindel et al., 1996). The developing human brain is uniquely vulnerable to toxic chemical exposures, and major windows of developmental vulnerability occur in the womb, during infancy and early childhood. Chemical exposure, even at low levels, can cause permanent brain injury during these fragile growth phases, where exposure during adulthood would have little or no adverse effects. Classified amongst other neurotoxic chemicals, fluoride, when administered at high levels, has the potential to cause neurotoxicity in adults and can result in negative impacts on memory and learning according to rodent study reports. Throughout the last several years, advances in scientific research have enabled expansion of what little understanding there is about the substance’s impact on children’s neurodevelopment.
Given that almost 65 years have passed since a daily intake of fluoride became the norm in the United States, there has grown to be a substantial amount of statistics to serve a basis for fluoride-based research. As of February 2015, roughly 50 studies have investigated the relationship between fluoride and human intelligence; in addition, approximately 33 studies have been conducted to explore fluoride’s affiliation with learning and memory in animals. The Fluoride Action Network, which is a non-for-profit organization against fluoride use, has constructed a database of these studies; out of the human investigations, 43 have found that elevated fluoride exposure is associated with reduced IQ, while 31 of the 33 animal studies have found that fluoride exposure impairs the learning and/or memory capacity of animals.
Exposure and Toxicity
Symptoms of acute oral fluoride intoxication in humans include severe nausea, vomiting, hyper-salivation, abdominal pain, and diarrhea. In severe or fatal cases, these symptoms are followed by convulsions, cardiac arrhythmias, and coma. In humans, acute toxic doses range from 1-5 mg/kg, where doses exceeding 15-30 mg/kg may be fatal. Acute intoxication side effects in experimental animals are similar to those observed in humans, however doses are determined by body weight. Acute oral LD50 values for fluoride compounds in laboratory animals range from 20 to 100 mg/kg (Mullenix, Denbesten, Schunior, & Kernan, 1995). According to research conducted on the physiological and toxicological characteristics of fluoride, a probable toxic dose of 5 mg fluoride per kilogram can be reached in a 10 kg, 1-year-old child after ingestion of approximately 50 mg fluoride tablets, 50 g of 1000 ppm fluoridated toothpaste, or 50 ml of 0.2% sodium fluoride rinse (Whitford, 1990). The study also suggests that ingestion of approximately twice these amounts can cause toxicity in a 5-year-old child, while mild gastrointestinal symptoms of acute intoxication may occur at doses as low as 1 mg/kg, or about one fifth of the probable toxic dose. Despite these intimidating statistics, there are potential benefits that come with the use of fluoride.
Chronic exposure to excessive fluoride is known to cause dental fluorosis, resulting in the discoloration and mottling of teeth, and skeletal fluorosis, which is the breakdown of bone-calcium in humans. Over exposure to fluoride during the first eight years of life can cause fluorosis, which is a cosmetic condition that affects the appearance of teeth resulting in stains and pits. Other effects, such as reproductive or developmental outcomes are still undergoing research
Acute Fluoride Exposure
There is logical reasoning behind the use of fluoride; experiments have been conducted to determine the relative effectiveness of various concentrations of fluoridated toothpaste in preventing dental caries in children and adolescents. In 2010, a study that had been published in the Cochrane Database of Systematic Reviews appeared particularly relevant. The study was composed of 74 trials, consisting of children six to sixteen years of age. A medley of researchers from the School of Dentistry at The University of Manchester in Manchester, UK organized the study by randomized-controlled trials and cluster-randomized controlled trials to compare the efficiency of high and low concentrations of fluoride toothpaste, as well as placebo toothpaste, over the course of one year (Walsh, et al., 2010). Out of 74 trials, preventive effect of tooth decay increased significantly with higher fluoride concentrations; the highest concentrations, ranging between 1000-1250 ppm was found to be the most effective, followed by the placebo. The results suggest the existence of benefits when using fluoride toothpaste in preventing caries in children and adolescents when compared to placebo, however, only fluoride concentrations of 1000 ppm and above were found to be effective. The relative caries preventive effects of fluoride toothpaste in concentrations lower than 550 ppm were found to have no statistical significance when compared to the placebo. In turn, it was proposed that there is some evidence of a dose response relationship in that the preventative effects increased as the fluoride concentration increased (Walsh, et al., 2010). Despite this finding, additional research on the benefits of fluoride use must be conducted to prove significant findings.
Chronic Fluoride Exposure
It has been discovered that lower IQ is found to strongly coincide with iodine deficiency (Zhai, Guo, Hu, Wang, & Zhu, 2003). The mechanism of lower IQ associated with iodine deficiency is related to certain neurotransmitters, enzymes, growth metabolism, and the development of the central nervous system. Recent investigations have shown reduction of learning-memory in rat offspring when mothers where administered high fluoride, low iodine or a combination of high fluoride and low iodine (Zhai et al., 2003). There are reports indicating that low iodine is directly related to oxidative stress. Oxidative stress is defined as an imbalance between higher cellular levels and reactive oxygen species (ROS), such as superoxide radical, hydrogen peroxide or nitric oxide, and cellular antioxidant defenses including superoxide dismutase. Brain tissue is particularly vulnerable to oxidative damage because of its high consumption of oxygen and the consequent generation of high quantities of free radicals.
As suggested by earlier research, excessive ingestion of fluoride can exert toxic effects on many tissues and organs, resulting in serious damage and pathological changes, where findings suggest there is a correlation between excessive exposure to fluoride and dysfunction of the central nervous system has been discovered (Gao et al., 2009). Scientists from Guiyang Medical University in China studied the mechanism of the decreased learning and memory in rats with chronic fluorosis, where both male and female young adult rats were administered either 5 or 50mg of fluoride via water supply for six months to evaluate levels of antioxidant capacity (T-AOC) and malondialdehyde (MDA) within brain tissue (Gao et al., 2009). According to the study, experimental rat brains subject to fluorosis had presented numerous histopathological changes, including demyelinization, a decrease in the number of Purkinje cells, thickening and disappearance of dendrites, swelling of mitochondria, and enlargement of endoplasmic reticulum in neurons.
It has been shown that fluoride can cross the blood brain barrier and accumulate in the brain of rats exposed to high fluoride concentrations. As a result, the severity of adverse effects of fluoride observed on behavior was directly correlated with the concentrations of fluoride ions in plasma and specific regions of the brain. The study proposed by researchers at Guiyang Medical University goes on to report that neurotoxic effects of long-term fluoride exposure results in oxidative stress, changes in the cholinergic nervous system, and decreased ability of learning and memory (Gao et al., 2009). Overall, the results suggest that in high doses, fluoride can induce deterioration of learning and memory capability in animals and in humans with chronic fluorosis. The research stresses that lower IQ and decreased mental capacity may occur as a result, particularly in children. In the particular experiment referenced, high fluoride intake induced a decreased capacity in learning and memory of rats.
In recent years, it has been recognized that higher concentrations of fluoride produce neuronal dysfunction by mechanisms involving elevated levels of such free radicals (Masaad & Klann, 2011). The scientists from Guiyang Medical University in China wanted to build upon their research by examining the relationship between fluoride and the production of free radicals. Under natural biological conditions, the level of ROS radical production is kept in a dynamic balance through levels of chemical elimination. However, under oxidative stress, high levels of free radicals are viewed as pathogenic factors, challenging the compensatory role of the antioxidant system. It was found that excessive production of ROS free radicals also appeared to play a role in diminishing cognitive ability processes such as learning and memory (Gao et al., 2009). High fluoride concentrations were found to be a free radical inducer, resulting in an increased level of lipid peroxidation, which may therefore have an important role in the pathogenesis of fluorosis.
In order to further evaluate the role of pathogenesis in fluorosis, levels of T-AOC and MDA influenced by fluorosis were examined. T-AOC is a useful index of the combined action of antioxidants in the body and MDA is an indicator for the products of lipid peroxidation (Gao et al., 2009). Lower levels of T-AOC and higher levels of MDA were observed in the brains of rats with the higher concentration of fluoride exposure, suggesting that fluoride induced oxidative stress occurred in the rat brains. In addition, decreased levels of T-AOC were found in the rat brains fed a lower concentration of fluoride whereas no significant changes in the level of MDA were found thereby indicating mild damage induced by the lower fluoride exposure (Gao et al., 2009).
Interestingly, the study found that a positive correlation was observed between the content of MDA and the prolonged time of escape latency in the rats fed with high fluoride intake. These results suggested that higher concentrations of fluoride could induce a decreased learning and memory capacity in which a possible mechanism might be connected to the high level of oxidative stress resulting from fluorosis (Gao et al., 2009). Since excessive amounts of fluoride can damage cellular membrane structure by lipid peroxidation, alteration in the membrane lipid composition could potentially influence the insertion or turnover of neuronal nicotinic acetylcholine receptors, the function of which is involved in memory and cognition, thus suggesting a close correlation between increased oxidative stress and altered behavior of the rats with fluorosis (Gao et al., 2009).
Influences of Fluoride Neurotoxicity
The hippocampus belongs to the limbic system and plays important role in the mediation of special navigation, memory, emotion, learning, sexual, and social behavior. Hippocampal cells greatly alter their activity in response to certain spatial correlates, particularly as an animal moves about in its environment. Hyperactivity and cognitive deficits are generally linked with hippocampal damage. In fact, the hippocampus is considered to be the central processor due to its integration of inputs from environment, memory, and motivational stimuli to produce behavioral responses, and to modify memory. A link has been found between fluoride exposures and developmental-behavioral disruptions occurring in rats, where the effect on behavior varies with the timing of central nervous system (CNS) development during exposure. The experiment consisted of both male and female rats that were administered daily fluoride concentrations of 100 ppm for a six-week duration, covering three developmental stages: prenatal, weanling, and adulthood. The fluoride was first administered to establish prenatal exposure and continued throughout postnatal days 17-19, which is a crucial developmental period where pyramidal cells of the hippocampus are forming. The chronic exposure continues throughout weanling and adult ages, when granule cells of the dentate gyrus of the hippocampus form (Zhai, Guo, Hu, Wang, & Zhu, 2003).
It has been suggested that drug responses in the brain correspond differently depending on which hormones are present at the time of drug exposure (Gao, et al., 2009). With this knowledge, researchers determined whether the interruption of normal brain development caused by fluoride toxicity results in sex-dependent responses. Considering the orbital cortex matures earlier in male primates than in females, developmental differences as such are commonly perceived accountable for the consequences of perinatal injuries appearing more frequently in males. This type of developmental difference might explain why, when testing both male and female rats on postnatal days 17-19, brief administrations of high fluoride concentrations affected only males. Gender-dependent dimorphic controls of growth are important to consider when evaluating fluoride distribution due to its affinity for calcium. Fluoride is deposited into mineral-forming bones more readily than in existing bone and thus, the rate of uptake by bone depends largely on stage of skeletal development (Gao, et al., 2009). As males experience greater and more prolonged growth spurts than females, it becomes more likely that their plasma fluoride appeals more to bone than to brain, perhaps explaining why longer exposures and higher plasma fluoride levels were needed in males to affect behavior. Fluoride’s tendency to seek developing bone may also explain why adult female rats had behavioral effects at a lower plasma fluoride concentration than did weanling female rats.
Behavioral changes common to weanling and adult exposures were different from those after prenatal exposures (Mullenix, et al., 1995). The study found that prenatal exposure dispersed many behaviors similar to those seen in drug induced hyperactivity, while weanling and adult exposures led to behavior-specific changes more related to cognitive deficits. Prenatally induced behavioral effects were unaccompanied by changes in body weight or elevated plasma fluoride levels. In contrast, the behavioral effects induced by weanling and adult exposures were always accompanied by elevated plasma fluoride levels. By opposing findings found in short-term fluoride kinetic studies that had been published in the Archives of Toxicology, it was concluded that effects on behavior was related directly to plasma fluoride levels and the fluoride accumulation in the brain (Guan, et al., 2000). The results suggest that impermeability of the adult blood-brain barrier does not directly relate to chronic exposure situations (Mullenix, et al., 1995).
Neurobehavioral and Developmental Toxicity
In a meta-analysis, researchers from Harvard School of Public Health (HSPH) and China Medical University in Shenyang combined 27 studies published throughout the last 23 years and found strong indications that fluoride may adversely affect cognitive development in children. Based on the findings, researchers suggest that this risk should not be ignored, and that more research on fluoride’s impact on the developing brain is warranted. The human studies, which are based on IQ examinations of over 11,000 children, provide compelling evidence that fluoride exposure during the early years of life can damage a child’s developing brain (Liu et al., 2014).
In order to determine the long-term effects of chronic fluoride exposure, subjects observed were children who lived in areas of China with high fluoride exposure. The exposed groups had access to drinking water with fluoride concentrations of up to 11.5 mg/L. That being said, in many cases concentrations were above the levels recommended or allowed in public drinking water (4.0 mg/L; U.S. EPA) in the United States. Children living in areas with high fluoride concentrations were found to have lower IQ scores than those who lived in low-exposure or control areas (Liu et al., 2014).
The results suggest that fluoride may be a developmental neurotoxicant that affects brain development at exposures much below those that can cause toxicity in adults. For neurotoxicants such as lead and methylmercury, adverse effects are associated with blood concentrations as low as 10 nmol/L; however, fluoride concentrations associated with high intakes from drinking water may exceed 1 mg/L, or 50 µmol/L, which is more than 1,000 times the levels of some other neurotoxicants that cause neurodevelopmental damage (Choi, Sun, Zhang, & Grandjean, 2012). In order to support the plausibility of these findings, researchers had rats exposed to 1 ppm of fluoridated water for one year, resulting in morphological alterations in the brain and increased levels of aluminum in brain tissue compared with controls (Liu et al., 2014).
In conclusion, these results support the possibility of adverse effects of fluoride exposures on children’s neurodevelopment. Future research should formally evaluate dose–response relations based on individual-level measures of exposure over time, as well as precise prenatal exposure assessments, and more extensive standardized measures of neurobehavioral performance.
In the U.S., about 70% of public water supplies are fluoridated, equating to approximately 185 million people, which is over half the number of people drinking artificially fluoridated water worldwide (ADA, 2005). Because many fluoride modalities are effective, inexpensive, readily available, and can be used in both private and public health settings, it is likely that fluoridation will continue to spread throughout the United States. According to the U.S. Public Health Service, the recommended range of optimal fluoridation of drinking water is set to be increased to 0.7-1.2 ppm by the year 2020. There is no need for additional fluoride to be added to the country’s water supply; it is well established that fluoride is not an essential nutrient. Despite studies suggesting beneficial use, there are other ways to combat tooth decay. Pit and fissure sealants, meticulous oral hygiene, and appropriate dietary practices contribute to caries prevention and control; however, the most effective and widely used approach is fluoride, and will likely remain this way until substantial evidence of it’s adverse effects on children are broadcasted.
ADA Fluoride Facts. (2005). Retrieved April 3, 2015, from http://www.ada.org/~/media/ADA/MemberCenter/FIles/fluoridation_facts.ashx
Choi, A. L., Sun, G., Zhang, Y., & Grandjean, P. (2012). Developmental
Fluoride Neurotoxicity: A Systematic Review and Meta-Analysis.
Environmental Health Perspectives, 120(10), 1362–1368.
Fluoride Action Network. (2012). Retrieved April 1, 2015, from
http://fluoridealert.org/faq/
From the Centers for Disease Control. Public Health Service report on
fluoride benefits and risks. (1991). Jama, 266(8), 1061-1062, 1066-1067.
Gao, Q., Liu, Y. J., & Guan, Z. Z. (2009). Decreased learning and memory
ability in rats with fluorosis: increased oxidative stress and reduced
cholinesterase activity in the brain. Fluoride, 42(4), 266-274.
Grandjean, P., & Landrigan, P. J. (2014). Neurobehavioural effects of
developmental toxicity. Lancet Neurology, 13(3), 330-338.
Guan, Z. Z., Xiao, K. Q., Zeng, X. Y., Long, Y. G., Cheng, Y. H., Jiang, S. F., &
Wang, Y. N. (2000). Changed cellular membrane lipid composition
and lipid peroxidation of kidney in rats with chronic fluorosis.
Archives of Toxicology, 74(10), 602-608.
Heindel, J. J., Bates, H. K., Price, C. J., Marr, M. C., Myers, C. B., & Schwetz,
B. A. (1996). Developmental toxicity evaluation of sodium fluoride
inistered to rats and rabbits in drinking water. Fundamental and
Applied Toxicology, 30(2), 162-177.
American Dental Association. (2014, January 1). Retrieved March 28, 2015,
from http://www.ada.org
Liu, F., Ma, J., Zhang, H., Liu, P., Liu, Y.-P., Xing, B., & Dang, Y.-H. (2014).
Fluoride exposure during development affects both cognition and
emotion in mice. Physiology &
Behavior, 124(0), 1-7.
Maddi, S. S., Tandon, S., & Aithal, K. S. (1999). Clinical evaluation of
sodium fluoride chewable tablets in dental caries. Indian Journal of
Dental Research,10(4), 146-149.
Massaad, C. A., & Klann, E. (2011). Reactive Oxygen Species in the
Regulation of Synaptic Plasticity and Memory. Antioxidants & Redox
Signaling, 14(10), 2013– 2054.
Mullenix, P. J., Denbesten, P. K., Schunior, A., & Kernan, W. J. (1995).
Neurotoxicity of sodium fluoride in rats. Neurotoxicology and
Teratology, 17(2), 169-177.
Mundy, W. (2009). Building a database of developmental neurotoxicants:
evidence from human and animal studies. Retrieved April 1, 2015,
from
http://www.epa.gov/ncct/toxcast/files/summit/48P Mundy TDAS.pdf
Walsh, T., Worthington, H. V., Glenny, A. M., Appelbe, P., Marinho, V. C., &
Shi, X. (2010). Fluoride toothpastes of different concentrations for
preventing dental caries in children and adolescents. Cochrane
Database of Systematic Reviews (1), Cd007868.
Whitford, G. M. (1990). The physiological and toxicological characteristics
of fluoride. Journal of Dental Research, 69, 539-549; discussion 556-
537.
Zhai, J. X., Guo, Z. Y., Hu, C. L., Wang, Q. N., & Zhu, Q. X. (2003). Studies on
fluoride concentration and cholinesterase activity in rat
hippocampus [Abstract]. Zhonghua Lao Dong Wei Sheng Zhi Ye Bing
Za Zhi, 21(2), 102-104.
Thank you very much, a very good contribution. We live in a Pharmageddon in which the collateral - called person - is used like a game character. Not to our advantage, of course. - Caries seems to be a infectious bacterial disease, nourished by glucose. What helps is xylitol, which kills caries bacteria. But never fluorine. Good luck!
/ᐠ._.ᐟ\
Wow, fluoride is straight up poison in every respect. That was about a 30 minute read! Very informative and detailed. Keep up the great work! we need more girls in the blockchain space!